Thank you for visiting nature.com. You are using a browser version with limited support for CSS. To obtain the best experience, we recommend you use a more up to date browser (or turn off compatibility mode in Internet Explorer). In the meantime, to ensure continued support, we are displaying the site without styles and JavaScript.
Nature Astronomy volume 7, pages 1317–1328 (2023 )Cite this article Zinc Selenide Plano-Convex Lenses
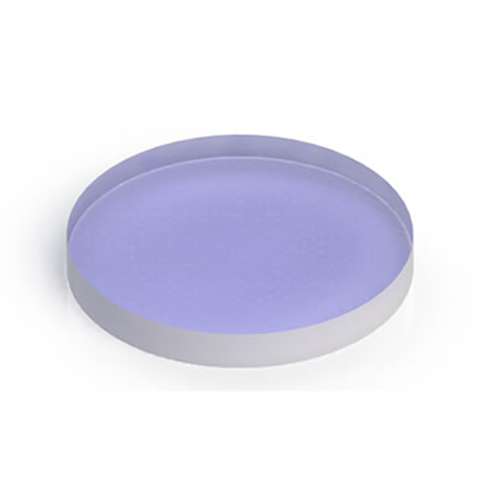
The critical first step in the search for life on exoplanets over the next decade is to determine whether rocky planets transiting small M-dwarf stars possess atmospheres and, if so, what processes sculpt them over time. Because of its broad wavelength coverage and improved resolution compared with previous instruments, spectroscopy with the James Webb Space Telescope (JWST) offers a new capability to detect and characterize the atmospheres of Earth-sized, M-dwarf planets. Here we use the JWST to independently validate the discovery of LHS 475 b, a warm (586 K), 0.99 Earth-radius exoplanet, interior to the habitable zone, and report a precise 2.9–5.3 μm transmission spectrum using the Near Infrared Spectrograph G395H instrument. With two transit observations, we rule out primordial hydrogen-dominated and cloudless pure methane atmospheres. Thus far, the featureless transmission spectrum remains consistent with a planet that has a high-altitude cloud deck (similar to Venus), a tenuous atmosphere (similar to Mars) or no appreciable atmosphere at all (akin to Mercury). There are no signs of stellar contamination due to spots or faculae. Our observations show that the JWST has the requisite sensitivity to constrain the secondary atmospheres of terrestrial exoplanets with absorption features <50 ppm, and that our current atmospheric constraints speak to the nature of the planet itself, rather than instrumental limits.
This is a preview of subscription content, access via your institution
Access Nature and 54 other Nature Portfolio journals
Get Nature+, our best-value online-access subscription
Receive 12 digital issues and online access to articles
Rent or buy this article
Prices vary by article type
Prices may be subject to local taxes which are calculated during checkout
The data used in this paper are from the JWST Cycle 1 General Observer Program 1981 and are publicly available on the Mikulski Archive for Space Telescopes (https://mast.stsci.edu). Fully reduced data products from this paper are available in the following Zenodo long-term public archive: https://doi.org/10.5281/zenodo.7925111. All additional data, such as intermediate data products and model outputs, are available upon request.
The codes used throughout this work for data analysis, atmospheric modelling and paper preparation are as follows: Astropy88,89, batman56, CHIMERA68,69, dynesty63, emcee57, Eureka!33, ExoCTK90, Forecaster38, IPython91, jwst47, Matplotlib92, NumPy93,94, PICASO67, POSEIDON80, PyMC395, SciPy96 and smarter76.
Delrez, L. et al. Early 2017 observations of TRAPPIST-1 with Spitzer. Mon. Not. R. Astron. Soc. 475, 3577–3597 (2018).
ADS Google Scholar
Diamond-Lowe, H., Berta-Thompson, Z., Charbonneau, D. & Kempton, E. M. R. Ground-based optical transmission spectroscopy of the small, rocky exoplanet GJ 1132b. Astron. J. 156, 42 (2018).
ADS Google Scholar
de Wit, J. et al. A combined transmission spectrum of the Earth-sized exoplanets TRAPPIST-1 b and c. Nature 537, 69–72 (2016).
ADS Google Scholar
de Wit, J. et al. Atmospheric reconnaissance of the habitable-zone Earth-sized planets orbiting TRAPPIST-1. Nat. Astron. 2, 214–219 (2018).
ADS Google Scholar
Zhang, Z., Zhou, Y., Rackham, B. V. & Apai, D. The near-infrared transmission spectra of TRAPPIST-1 planets b, c, d, e, f, and g and stellar contamination in multi-epoch transit spectra. Astron. J. 156, 178 (2018).
ADS Google Scholar
Diamond-Lowe, H., Charbonneau, D., Malik, M., Kempton, E. M. R. & Beletsky, Y. Optical transmission spectroscopy of the terrestrial exoplanet LHS 3844b from 13 ground-based transit observations. Astron. J. 160, 188 (2020).
ADS Google Scholar
Edwards, B. et al. Hubble WFC3 spectroscopy of the habitable-zone super-Earth LHS 1140 b. Astron. J. 161, 44 (2021).
ADS Google Scholar
Diamond-Lowe, H., Mendonça, J. M., Charbonneau, D. & Buchhave, L. A. Ground-based optical transmission spectroscopy of the nearby terrestrial exoplanet LTT 1445Ab. Astron. J. 165, 169 (2023).
ADS Google Scholar
Kreidberg, L. et al. Absence of a thick atmosphere on the terrestrial exoplanet LHS 3844b. Nature 573, 87–90 (2019).
ADS Google Scholar
Swain, M. R. et al. Detection of an atmosphere on a rocky exoplanet. Astron. J. 161, 213 (2021).
ADS Google Scholar
Mugnai, L. V. et al. ARES. V. No evidence for molecular absorption in the HST WFC3 spectrum of GJ 1132 b. Astron. J. 161, 284 (2021).
ADS Google Scholar
Libby-Roberts, J. E. et al. The featureless HST/WFC3 transmission spectrum of the rocky exoplanet GJ 1132b: no evidence for a cloud-free primordial atmosphere and constraints on starspot contamination. Astron. J. 164, 59 (2022).
ADS Google Scholar
Seager, S. & Deming, D. On the method to infer an atmosphere on a tidally locked super Earth exoplanet and upper limits to GJ 876d. Astrophys. J. 703, 1884–1889 (2009).
ADS Google Scholar
Mansfield, M. et al. Identifying atmospheres on rocky exoplanets through inferred high albedo. Astrophys. J. 886, 141 (2019).
ADS Google Scholar
Morley, C. V., Kreidberg, L., Rustamkulov, Z., Robinson, T. & Fortney, J. J. Observing the atmospheres of known temperate Earth-sized planets with JWST. Astrophys. J. 850, 121 (2017).
ADS Google Scholar
Fujii, Y. et al. Exoplanet biosignatures: observational prospects. Astrobiology 18, 739–778 (2018).
ADS Google Scholar
Batalha, N. E., Lewis, N. K., Line, M. R., Valenti, J. & Stevenson, K. Strategies for constraining the atmospheres of temperate terrestrial planets with JWST. Astrophys. J. Lett. 856, L34 (2018).
ADS Google Scholar
Exoplanet Science Strategy (National Academies, 2018).
Lustig-Yaeger, J., Meadows, V. S. & Lincowski, A. P. The detectability and characterization of the TRAPPIST-1 exoplanet atmospheres with JWST. Astron. J. 158, 27 (2019).
ADS Google Scholar
Garcia-Sage, K., Glocer, A., Drake, J. J., Gronoff, G. & Cohen, O. On the magnetic protection of the atmosphere of Proxima Centauri b. Astrophys. J. Lett. 844, L13 (2017).
ADS Google Scholar
Airapetian, V. S. et al. Impact of space weather on climate and habitability of terrestrial-type exoplanets. Int. J. Astrobiol. 19, 136–194 (2020).
ADS Google Scholar
Segura, A. et al. Biosignatures from Earth-like planets around M dwarfs. Astrobiology 5, 706–725 (2005).
ADS Google Scholar
Tian, F. Atmospheric escape from Solar System terrestrial planets and exoplanets. Annu. Rev. Earth Planet. Sci. 43, 459–476 (2015).
ADS Google Scholar
Luger, R. & Barnes, R. Extreme water loss and abiotic O2 buildup on planets throughout the habitable zones of M dwarfs. Astrobiology 15, 119–143 (2015).
ADS Google Scholar
Jakobsen, P. et al. The Near-Infrared Spectrograph (NIRSpec) on the James Webb Space Telescope. I. Overview of the instrument and its capabilities. Astron. Astrophys. 661, A80 (2022).
Birkmann, S. M. et al. The Near-Infrared Spectrograph (NIRSpec) on the James Webb Space Telescope. IV. Capabilities and predicted performance for exoplanet characterization. Astron. Astrophys. 661, A83 (2022).
Ricker, G. R. et al. Transiting Exoplanet Survey Satellite (TESS). J. Astron. Telesc. Instrum. Syst. 1, 014003 (2015).
ADS Google Scholar
Guerrero, N. M. et al. The TESS Objects of Interest Catalog from the TESS Prime Mission. Astrophys. J. Suppl. Ser. 254, 39 (2021).
ADS Google Scholar
Ment, K. & Charbonneau, D. The occurrence rate of terrestrial planets orbiting nearby mid-to-late M dwarfs from TESS Sectors 1–42. Astron. J. 165, 265 (2023).
ADS Google Scholar
Stassun, K. G. et al. The Revised TESS Input Catalog and Candidate Target List. Astron. J. 158, 138 (2019).
ADS Google Scholar
Kasting, J. F., Whitmire, D. P. & Reynolds, R. T. Habitable zones around main sequence stars. Icarus 101, 108–128 (1993).
ADS Google Scholar
Ment, K. et al. LHS 475 b: A Venus-sized planet orbiting a nearby M dwarf. Preprint at https://arxiv.org/abs/2304.01920 (2023).
Bell, T. J. et al. Eureka!: An end-to-end pipeline for JWST time-series observations. J. Open Source Softw. 7, 4503 (2022).
ADS Google Scholar
Rustamkulov, Z. et al. Early release science of the exoplanet WASP-39b with JWST NIRSpec PRISM. Nature 614, 659–663 (2023).
Kirk, J. et al. LRG-BEASTS III: ground-based transmission spectrum of the gas giant orbiting the cool dwarf WASP-80. Mon. Not. R. Astron. Soc. 474, 876–885 (2018).
ADS Google Scholar
Kirk, J. et al. LRG-BEASTS: transmission spectroscopy and retrieval analysis of the highly inflated Saturn-mass planet WASP-39b. Astron. J. 158, 144 (2019).
ADS Google Scholar
Kirk, J. et al. ACCESS and LRG-BEASTS: a precise new optical transmission spectrum of the ultrahot Jupiter WASP-103b. Astron. J. 162, 34 (2021).
ADS Google Scholar
Chen, J. & Kipping, D. Probabilistic forecasting of the masses and radii of other worlds. Astrophys. J. 834, 17 (2017).
ADS Google Scholar
Luque, R. & Pallé, E. Density, not radius, separates rocky and water-rich small planets orbiting M dwarf stars. Science 377, 1211–1214 (2022).
ADS Google Scholar
Magic, Z., Chiavassa, A., Collet, R. & Asplund, M. The Stagger-grid: a grid of 3D stellar atmosphere models. IV. Limb darkening coefficients. Astron. Astrophys. 573, A90 (2015).
ADS Google Scholar
Rackham, B. V., Apai, D. & Giampapa, M. S. The transit light source effect: false spectral features and incorrect densities for M-dwarf transiting planets. Astrophys. J. 853, 122 (2018).
ADS Google Scholar
Trotta, R. Bayes in the sky: Bayesian inference and model selection in cosmology. Contemp. Phys. 49, 71–104 (2008).
ADS Google Scholar
Kreidberg, L. et al. Clouds in the atmosphere of the super-Earth exoplanet GJ1214b. Nature 505, 69–72 (2014).
ADS Google Scholar
Tremblay, L. et al. The detectability and constraints of biosignature gases in the near- and mid-infrared from transit transmission spectroscopy. Astron. J. 159, 117 (2020).
ADS Google Scholar
Medina, A. A., Winters, J. G., Irwin, J. M. & Charbonneau, D. Flare rates, rotation periods, and spectroscopic activity indicators of a volume-complete sample of mid- to late-M dwarfs within 15 pc. Astrophys. J. 905, 107 (2020).
ADS Google Scholar
Koll, D. D. B. et al. Identifying candidate atmospheres on rocky M dwarf planets via eclipse photometry. Astrophys. J. 886, 140 (2019).
ADS Google Scholar
Bushouse, H. et al. JWST calibration pipeline. Zenodo https://doi.org/10.5281/zenodo.7325378 (2022).
JWST Transiting Exoplanet Community Early Release Science Team. Identification of carbon dioxide in an exoplanet atmosphere. Nature 614, 649–652 (2023).
Espinoza, N. et al. Spectroscopic time-series performance of JWST/NIRSpec from commissioning observations. Publ. Astron. Soc. Pac. 135, 018002 (2023).
Rustamkulov, Z., Sing, D. K., Liu, R. & Wang, A. Analysis of a JWST NIRSpec lab time series: characterizing systematics, recovering exoplanet transit spectroscopy, and constraining a noise floor. Astrophys. J. Lett. 928, L7 (2022).
ADS Google Scholar
Laginja, I. & Wakeford, H. R. ExoTiC-ISM: a Python package for marginalised exoplanet transit parameters across a grid of systematic instrument models. J. Open Source Softw. 5, 2281 (2020).
ADS Google Scholar
Laginja, I. & Wakeford, H. R. Exotic-ism v2.0.0. Zenodo https://doi.org/10.5281/zenodo.3923986 (2020).
Wakeford, H. & Grant, D. Exo-tic/exotic-ld: Exotic-ld v2.1 zenodo release. Zenodo https://doi.org/10.5281/zenodo.6809899 (2022).
Claret, A. A new non-linear limb-darkening law for LTE stellar atmosphere models. Calculations for −5.0 <= log[M/H] <= +1, 2000 K <= Teff <= 50000 K at several surface gravities. Astron. Astrophys. 363, 1081–1190 (2000).
ADS Google Scholar
Sing, D. K. Stellar limb-darkening coefficients for CoRot and Kepler. Astron. Astrophys. 510, A21 (2010).
ADS Google Scholar
Kreidberg, L. batman: basic transit model calculation in Python. Publ. Astron. Soc. Pac. 127, 1161 (2015).
ADS Google Scholar
Foreman-Mackey, D., Hogg, D. W., Lang, D. & Goodman, J. emcee: the MCMC hammer. Publ. Astron. Soc. Pac. 125, 306 (2013).
ADS Google Scholar
Kruse, E., Agol, E., Luger, R. & Foreman-Mackey, D. Detection of hundreds of new planet candidates and eclipsing binaries in K2 campaigns 0–8. Astrophys. J. Suppl. Ser. 244, 11 (2019).
ADS Google Scholar
Gaia Collaboration et al. Gaia Early Data Release 3. Summary of the contents and survey properties. Astron. Astrophys. 649, A1 (2021).
Sandford, E. & Kipping, D. Know the planet, know the star: precise stellar densities from Kepler transit light curves. Astron. J. 154, 228 (2017).
ADS Google Scholar
Rigby, J. et al. The science performance of JWST as characterized in commissioning. Publ. Astron. Soc. Pac. 135, 048001 (2023).
Husser, T. O. et al. A new extensive library of PHOENIX stellar atmospheres and synthetic spectra. Astron. Astrophys. 553, A6 (2013).
Speagle, J. S. dynesty: a dynamic nested sampling package for estimating Bayesian posteriors and evidences. Mon. Not. R. Astron. Soc. 493, 3132–3158 (2020).
ADS Google Scholar
Skilling, J. In Bayesian Inference and Maximum Entropy Methods in Science and Engineering: 24th International Workshop on Bayesian Inference and Maximum Entropy Methods in Science and Engineering American Institute of Physics Conference Series Vol. 735 (eds Fischer, R. et al.) 395–405 (American Institute of Physics, 2004).
Turbet, M., Ehrenreich, D., Lovis, C., Bolmont, E. & Fauchez, T. The runaway greenhouse radius inflation effect. An observational diagnostic to probe water on Earth-sized planets and test the habitable zone concept. Astron. Astrophys. 628, A12 (2019).
ADS Google Scholar
Moran, S. E., Hörst, S. M., Batalha, N. E., Lewis, N. K. & Wakeford, H. R. Limits on clouds and hazes for the TRAPPIST-1 planets. Astron. J. 156, 252 (2018).
ADS Google Scholar
Batalha, N. E. et al. The precision of mass measurements required for robust atmospheric characterization of transiting exoplanets. Astrophys. J. Lett. 885, L25 (2019).
ADS Google Scholar
Line, M. R. & Yung, Y. L. A systematic retrieval analysis of secondary eclipse spectra. III. Diagnosing chemical disequilibrium in planetary atmospheres. Astrophys. J. 779, 3 (2013).
ADS Google Scholar
Line, M. R., Knutson, H., Wolf, A. S. & Yung, Y. L. A systematic retrieval analysis of secondary eclipse spectra. II. A uniform analysis of nine planets and their C to O ratios. Astrophys. J. 783, 70 (2014).
ADS Google Scholar
McBride, B. J. & Gordon, S. Computer Program for Calculation of Complex Chemical Equilibrium Compositions and Applications II. User’s Manual and Program Description Technical Report (NASA, 1996).
Guillot, T. On the radiative equilibrium of irradiated planetary atmospheres. Astron. Astrophys. 520, A27 (2010).
ADS MATH Google Scholar
Freedman, R. S., Marley, M. S. & Lodders, K. Line and mean opacities for ultracool dwarfs and extrasolar planets. Astrophys. J. Suppl. Ser. 174, 504–513 (2008).
ADS Google Scholar
Freedman, R. S. et al. Gaseous mean opacities for giant planet and ultracool dwarf atmospheres over a range of metallicities and temperatures. Astrophys. J. Suppl. Ser. 214, 25 (2014).
ADS Google Scholar
Batalha , N. , Freedman , R. , Lupu , R. & Marley , M. Resampled opacity database for PICASO v2.Zenodo https://doi.org/10.5281/zenodo.3759675 (2020).
Lustig-Yaeger, J. The Detection, Characterization, and Retrieval of Terrestrial Exoplanet Atmospheres. PhD thesis, Univ. Washington (2020); https://www.proquest.com/dissertations-theses/detection-characterization-retrieval-terrestrial/docview/2457714855/se-2?accountid=11752
Lustig-Yaeger, J. et al. Hierarchical Bayesian atmospheric retrieval modeling for population studies of exoplanet atmospheres: a case study on the habitable zone. Astron. J. 163, 140 (2022).
ADS Google Scholar
Meadows, V. S. & Crisp, D. Ground-based near-infrared observations of the Venus nightside: the thermal structure and water abundance near the surface. J. Geophys. Res. 101, 4595–4622 (1996).
ADS Google Scholar
Robinson, T. D. A theory of exoplanet transits with light scattering. Astrophys. J. 836, 236 (2017).
ADS Google Scholar
Gordon, I. E. et al. The HITRAN2016 molecular spectroscopic database. J. Quant. Spectrosc. Radiat. Transf. 203, 3–69 (2017).
ADS Google Scholar
MacDonald, R. J. & Madhusudhan, N. HD 209458b in new light: evidence of nitrogen chemistry, patchy clouds and sub-solar water. Mon. Not. R. Astron. Soc. 469, 1979–1996 (2017).
ADS Google Scholar
Kaltenegger, L. et al. The white dwarf opportunity: robust detections of molecules in Earth-like exoplanet atmospheres with the James Webb Space Telescope. Astrophys. J. Lett. 901, L1 (2020).
ADS Google Scholar
Lin, Z., MacDonald, R. J., Kaltenegger, L. & Wilson, D. J. Differentiating modern and prebiotic Earth scenarios for TRAPPIST-1e: high-resolution transmission spectra and predictions for JWST. Mon. Not. R. Astron. Soc. 505, 3562–3578 (2021).
ADS Google Scholar
MacDonald, R. J. & Lewis, N. K. TRIDENT: a rapid 3D radiative-transfer model for exoplanet transmission spectra. Astrophys. J. 929, 20 (2022).
ADS Google Scholar
Feroz, F., Hobson, M. P. & Bridges, M. MULTINEST: an efficient and robust Bayesian inference tool for cosmology and particle physics. Mon. Not. R. Astron. Soc. 398, 1601–1614 (2009).
ADS Google Scholar
Buchner, J. et al. X-ray spectral modelling of the AGN obscuring region in the CDFS: Bayesian model selection and catalogue. Astron. Astrophys. 564, A125 (2014).
Benneke, B. & Seager, S. Atmospheric retrieval for super-Earths: uniquely constraining the atmospheric composition with transmission spectroscopy. Astrophys. J. 753, 100 (2012).
ADS Google Scholar
Aitchison, J. The Statistical Analysis of Compositional Data Monographs on Statistics and Applied Probability (Springer, 1986).
Astropy Collaboration et al. Astropy: a community Python package for astronomy. Astron. Astrophys. 558, A33 (2013).
Astropy Collaboration et al. The Astropy project: building an open-science project and status of the v2.0 core package. Astron. J. 156, 123 (2018).
ADS Google Scholar
Bourque, M. et al. The exoplanet characterization toolkit (exoctk). Zenodo https://doi.org/10.5281/zenodo.4556063 (2021).
Pérez, F. & Granger, B. E. IPython: a system for interactive scientific computing. Comput. Sci. Eng. 9, 21–29 (2007).
Hunter, J. D. Matplotlib: a 2D graphics environment. Comput. Sci. Eng. 9, 90–95 (2007).
van der Walt, S., Colbert, S. C. & Varoquaux, G. The NumPy array: a structure for efficient numerical computation. Comput. Sci. Eng. 13, 22–30 (2011).
Harris, C. R. et al. Array programming with NumPy. Nature 585, 357–362 (2020).
ADS Google Scholar
Salvatier, J., Wieckiâ, T. V. & Fonnesbeck, C. PyMC3: Python probabilistic programming framework. Astrophysics Source Code Library ascl:1610.016 (2016).
Virtanen, P. et al.SciPy 1.0: fundamental algorithms for scientific computing in Python.Nat.Methods 17, 261–272 (2020).
This work is based in part on observations made with the NASA/ESA/CSA JWST. The data were obtained from the Mikulski Archive for Space Telescopes (MAST) at the Space Telescope Science Institute (STScI), which is operated by the Association of Universities for Research in Astronomy, Inc., under NASA contract NAS 5-03127 for JWST. These observations are associated with programme 1981. Support for programme 1981 was provided by NASA through a grant from the STScI. This paper includes data collected with the TESS mission, obtained from the MAST data archive at the STScI. Funding for the TESS mission is provided by the NASA Explorer Program. The material is based on work supported by NASA under award number 80GSFC21M0002. This material is based upon work performed as part of NASA’s CHAMPs team, supported by NASA under Grant No. 80NSSC21K0905 issued through the Interdisciplinary Consortia for Astrobiology Research (ICAR) programme. R.J.M. is an NHFP Sagan Fellow.
Johns Hopkins APL, Laurel, MD, USA
Jacob Lustig-Yaeger, E. M. May, Kevin B. Stevenson, L. C. Mayorga & Kristin S. Sotzen
Department of Physics and Astronomy, Johns Hopkins University, Baltimore, MD, USA
Guangwei Fu & David K. Sing
Center for Astrophysics, Harvard & Smithsonian, Cambridge, MA, USA
Kevin N. Ortiz Ceballos, Mercedes López-Morales & Jéa I. Adams Redai
Lunar and Planetary Laboratory, University of Arizona, Tucson, AZ, USA
University of Maryland, Baltimore County, MD, USA
NASA Goddard Space Flight Center, Greenbelt, MD, USA
Sarah Peacock & Ethan Kruse
Department of Physics, Imperial College London, London, UK
Department of Astronomy, University of Michigan, Ann Arbor, MI, USA
Department of Earth and Planetary Sciences, Johns Hopkins University, Baltimore, MD, USA
David K. Sing, Katherine A. Bennett, Junellie Gonzalez-Quiles & Zafar Rustamkulov
Space Telescope Science Institute, Baltimore, MD, USA
Carnegie Earth and Planets Laboratory, Washington DC, USA
NASA Ames Research Center, Moffett Field, CA, USA
Department of Astronomy, University of Maryland, College Park, MD, USA
Center for Research and Exploration in Space Science and Technology, NASA/GSFC, Greenbelt, MD, USA
Department of Physics, Utah Valley University, Orem, UT, USA
School of Physics, HH Wills Physics Laboratory, University of Bristol, Bristol, UK
You can also search for this author in PubMed Google Scholar
You can also search for this author in PubMed Google Scholar
You can also search for this author in PubMed Google Scholar
You can also search for this author in PubMed Google Scholar
You can also search for this author in PubMed Google Scholar
You can also search for this author in PubMed Google Scholar
You can also search for this author in PubMed Google Scholar
You can also search for this author in PubMed Google Scholar
You can also search for this author in PubMed Google Scholar
You can also search for this author in PubMed Google Scholar
You can also search for this author in PubMed Google Scholar
You can also search for this author in PubMed Google Scholar
You can also search for this author in PubMed Google Scholar
You can also search for this author in PubMed Google Scholar
You can also search for this author in PubMed Google Scholar
You can also search for this author in PubMed Google Scholar
You can also search for this author in PubMed Google Scholar
You can also search for this author in PubMed Google Scholar
You can also search for this author in PubMed Google Scholar
You can also search for this author in PubMed Google Scholar
You can also search for this author in PubMed Google Scholar
You can also search for this author in PubMed Google Scholar
You can also search for this author in PubMed Google Scholar
All authors played a substantial role in one or more of the following: development of the original proposal, management of the project, observation planning, analysis of the data, theoretical modelling and preparation of this paper. J.L.-Y., G.F., K.B.S., E.M.M., K.N.O.C., S.E.M., S.P., M.L.-M., R.J.M. and J.K. made notable contributions to the figures and text of the paper. K.B.S., J.L.-Y., M.L.-M. and D.K.S. provided general programme leadership and management. K.B.S., J.L.-Y., E.M.M., S.E.M., D.K.S., K.S.S., N.E.B., J.D.L. and H.R.W. made important contributions to the design of the programme. K.B.S. created the observing plan with input from the team. L.C.M., J.K., D.K.S., K.S.S., J.A.V., J.I.A.R., M.K.A., N.E.B., K.A.B., J.G.-Q., E.K., J.D.L., Z.R. and H.R.W. contributed to the preparation of the paper.
Correspondence to Jacob Lustig-Yaeger or Guangwei Fu.
The authors declare no competing interests.
Nature Astronomy thanks Hannah Diamond-Lowe and the other, anonymous, reviewer(s) for their contribution to the peer review of this work.
Publisher’s note Springer Nature remains neutral with regard to jurisdictional claims in published maps and institutional affiliations.
Comparison of our 30 closest matching PHOENIX models (\({{\chi }^{2}}_{\nu } <\) 50) to all available archival photometry of LHS 475 from the VizieR Photometry Viewer. These models have Teff = 3300+80−30 K, log(g) = 5.2 ± 0.5 g/cm2, M = 0.262 M⊙ . The error bars are 1-σ standard deviations.
Left: A 3.36’ × 3.36’ DSS image centered on LHS 475 taken 1999 June 20. The red circle depicts the star’s J2000 position per Simbad, whereas the blue circle indicates the star’s position for the JWST observations in September of 2022. We see no indication of a background star at the 2022 position that could be the source of the observed transit signal. For reference, NIRSpec’s field of view is 1.6 × 1.6 pixels on this image. Right: Sensitivity curve of LHS 475 from a NIRSpec target acquisition image (upper right) spanning 1.6" × 1.6", using the SUB32 subarray and CLEAR filter. The sensitivity curve and visual inspection of the image show no contamination sources (down to Δmag=3) to within 0.1 arcsec, which corresponds to a distance of 1.25 AU.
Blue circles: FIREFly; red squares: Eureka!; yellow triangles: Tiberius. The error bars are 1-σ standard deviations. For plotting purposes, we bin the transmission spectra to a resolution of ~ 40 nm. We see excellent agreement between reductions from 2.8 - 4.5 μm. At > 4.5 μm, the binned spectra begin to diverge, though the unbinned data (not shown) are all consistent within 1σ.
Prior PDFs are in orange and posterior PDFs are in dark blue. The flat spectrum reveals a consistent spot (and faculae) coverage along the transit chord compared to the full stellar disk.
LHS 475 b’s binned spectrum is represented by black circles. The error bars are 1-σ standard deviations showing the uncertainties in the transit depth and width of each wavelength bin. Our data weakly rule out Earth composition (blue solid), clear Titan composition (orange solid), and clear Venus composition atmospheres (yellow solid). However, the data are all consistent within error to that of a hazy Titan composition with a haze-top at 0.01 mbar (dotted orange), a cloudy Venus composition with a cloud-top at 1 mbar (dotted yellow), and a Mars composition atmosphere (red solid), as well as that of an airless body, like Mercury (grey dotted line).
The upper right axis shows the transmission spectrum data with 1-? standard deviation error bars along with 1σ and 3σ envelopes around the median retrieved spectrum, which corresponds to the multidimensional posterior PDF projected onto the observed spectrum. Disfavored atmospheres are thick (large P0), hot (large T0), and composed primarily of light molecules (low μ).
Two retrievals with different prior treatments for the atmospheric composition are overplotted: centered log-ratio (CLR) transformed abundances with a priori unknown composition (green); and log-uniform abundances assuming an N2-dominated atmosphere (orange). Statistical 2σ upper and lower limits are annotated (or ‘N/A’ if unconstrained). Both retrievals rule out H2-dominated atmospheres. The log- uniform retrieval finds upper limits on H2O, CH4, CO2, and CO due to the assumption that N2 dominates the atmosphere, while the agnostic CLR treatment does not find upper limits for their abundances. For clarity in viewing upper limits, we switch from a logarithmic to linear x-axis at a mixing ratio of 10%. The probability densities for the linear histogram bins are renormalized to match the probability density of the nearest logarithmic bin left of the 10% boundary.
The units are: Rp, ref (R⊕ ), g (cm s−2), Psurf (bar), and T (K). The inset shows the corresponding retrieved transmission spectrum model (1σ and 2σ confidence regions) compared to the NIRSpec G395H observations. The error bars are 1-σ standard deviations. The solution rules out H2-dominated atmospheres (to > 5σ) and thick atmospheres (Psurf ≳ 10 mbar) dominated by CH4 (to 3σ).
Top: Eureka! spectrophotometric precision at the native pixel resolution, compared to expected noise levels for both events. The expected noise level, as well as 1.25 × and 2 × the expected noise are shown as grey lines, these have been smoothed to the resolution of the final transit spectrum for visualization purposes. Squares denote columns which are greater than 1.5 × the expected noise level in both transits, dark blue circles denote columns which are greater than 1.5 × the expected noise level in only one transit. These columns are flagged and not used to generate the final transmission spectrum. Bottom: Comparison of uncertainty on planet radius derived from light curves fit at the native pixel resolution and fitting of pre- binned light curves. The y-axis is in parts per thousand. We find little to no difference in the uncertainty, suggesting that our 1/f correction is sufficient to address the column-column variances.
Panel (a) illustrates that the white light curve residuals from two of the analyses exhibit some correlated noise at timescales of < 5 minutes ( < 35 integrations). This is likely due to uncorrected 1/f noise from the thermal cycling of on-board heaters [61, Section 4.5.3]. At longer timescales ( > 120 integrations, > 18 minutes), the Eureka! pipeline returns to the expected standard error with RMS values below 10 ppm. The Tiberius reduction did not sum the flux across both detectors and was not used for this noise floor analysis. The spectroscopic RMS values in panels (b)–(d) are more consistent with the standard error, thus confirming that the spectroscopic light curves are dominated by white noise.
Springer Nature or its licensor (e.g. a society or other partner) holds exclusive rights to this article under a publishing agreement with the author(s) or other rightsholder(s); author self-archiving of the accepted manuscript version of this article is solely governed by the terms of such publishing agreement and applicable law.
Lustig-Yaeger, J., Fu, G., May, E.M. et al. A JWST transmission spectrum of the nearby Earth-sized exoplanet LHS 475 b. Nat Astron 7, 1317–1328 (2023). https://doi.org/10.1038/s41550-023-02064-z
DOI: https://doi.org/10.1038/s41550-023-02064-z
Anyone you share the following link with will be able to read this content:
Sorry, a shareable link is not currently available for this article.
Provided by the Springer Nature SharedIt content-sharing initiative
The Astronomy and Astrophysics Review (2023)
Nature Astronomy (Nat Astron) ISSN 2397-3366 (online)
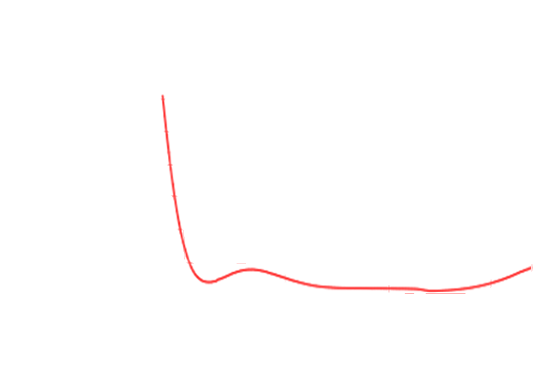
Swir Sign up for the Nature Briefing newsletter — what matters in science, free to your inbox daily.